News Story
Jewell Lab Explores Techniques to Tailor MS Therapy Design
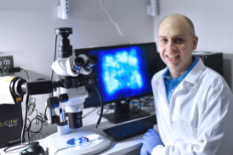
Minta Martin Professor of Engineering Christopher Jewell
University of Maryland bioengineers are taking a closer look at how changes to the biophysical properties of candidate nanotherapeutics might be leveraged to create more precision immunotherapies to treat multiple sclerosis (MS). In particular, Minta Martin Professor of Engineering Christopher Jewell and members of his Immune Engineering Lab are building immunotherapeutics entirely from immune signals, and carefully designing the sequence of these signals to change properties such as size and charge. The team has shown how these properties – not just the actual immune signals – alter how immune cells respond to the therapeutics and the outcomes. Their findings are now available in American Chemical Society journal, Nano Letters.
Nearly 2.5 million people worldwide have been diagnosed with MS, an autoimmune disease that causes the body’s immune system to wrongfully attack myelin, the insulation that surrounds and protects nerve fibers in the brain and spinal cord. When this happens, nerve fibers and cells are damaged, leading to a loss of motor function and other neurological complications that greatly impact the patient’s quality of life.
Current therapies for MS non-specifically decrease the activity of the immune system to restrain the detrimental “attack,” but at a cost that leaves MS patients vulnerable to certain infections or illnesses, including some cancers.
Recently, Jewell and his team have been working with specially designed nanomaterials built entirely from immune signals; they hope this unique design can be used to reprogram how the immune system responds to the body’s own healthy cells – or, “self” cells – in the brain and spinal cord during MS.
Studies have shown that some of the body’s typical defense mechanisms against infection – such as those known as toll-like receptors (TLRs) – go haywire during autoimmune disease and are, in fact, overactive in MS. Jewell and his team decided to target these pathways to block inflammation. Their hope is that, by eliminating harmful cues while delivering helpful myelin self-molecules, they might create an opportunity to retrain self-reactive T cells that attack myelin to instead become regulatory T cells. These special immune cells could enable more specific control of the cells that do damage during MS or other autoimmune diseases, without suppressing the function of normal cells.
In the human body, the immune system detects antigens – molecules that are present on all cells and vary according to the type of cell – to distinguish the body’s own “self cells” from foreign cells. When functioning properly, the body’s immune system recognizes self cells by their self-antigens; this, in turn, means that foreign substances — such as bacteria, toxins, or a virus — stick out like a sore thumb, enabling the immune system to wage a specific attack and elimination.
Antigen-presenting cells (APCs) play a significant role in mediating immune response by recognizing and processing antigens. Realizing this, Jewell and his team have investigated ways to deliver both self-antigens and modulatory immune cues to APCs to help teach the immune system to halt the attack on myelin.
While this approach offers promise, antigen-specific therapies have not yet been successful in patients, in part due to the difficulty of targeting immune cues to spatially restricted receptors on and within immune cells. Whether the immune system triggers an inflammatory response or a tolerant response against a self-antigen depends on how it perceives the self-antigens. Because of this, technologies that enable more precise control over immune signal integration would represent a new milestone for autoimmune therapies.
The key is recognizing that outcomes during immunotherapy are impacted not only by the specific therapeutic signals, but also by the biophysical forms in which signals are delivered. Unfortunately, the links between nanomaterial design, biophysical properties, and immune regulation are poorly defined.
“This goal will be enabled by deeper understanding of not just the role of the signals in immunotherapies, but also the role of how the signals are displayed,” Jewell said. “Properties such as nanoparticle size, charge, and strength of interaction are all important in immune signals integration. Here, we’ve been able to study these biophysical properties more directly since we are building nanoparticles entirely from immune signals, without other conventional polymer or lipid carriers.”
In this new paper – led by Eugene Froimchuk, Clark Doctoral Fellow and National Science Foundation Graduate Fellow in the Jewell Lab – the team made some exciting findings along these lines. In particular, they showed that the charge designed into myelin antigens used in their therapeutics controlled how tightly the components binded tolerizing immune cues. As a result, the interactions with immune cells were altered, leading to control over the type of immune cells generated. In particular, the best designs efficiently controlled myelin-specific regulatory immune cells important in combating autoimmune diseases such as multiple sclerosis. The team’s belief is that this granular understanding of nanomaterial-immune interactions will contribute to rational design rules for immunotherapies that selectively promote tolerance without impacting normal immune function needed to combat infection.
In addition to graduate fellow Eugene Froimchuk, other authors on the team include BIOE postdoctoral researcher Robert “Smitty” Oakes, BIOE Assistant Research Scientist Senta Kapnick, and BIOE Faculty Specialist Alexis Yanes.
In addition to his BIOE appointment, Jewell is a research biologist with the U.S. Department of Veterans Affairs and a faculty member with the University of Maryland’s Robert E. Fischell Institute for Biomedical Devices.
This work was supported in part by the U.S. Department of Veterans Affairs and the National Institutes of Health. In addition, Froimchuk’s work was supported in part by a National Science Foundation Graduate Research Fellowship Program Grant and the A. James Clark School of Engineering’s Clark Doctoral Fellowship.
University of Maryland BioWorkshop core facility manager Sijie Hao contributed training and technical assistance.
Full text of the paper titled “Biophysical Properties of Self-Assembled Immune Signals Impact Signal Processing and the Nature of Regulatory Immune Function” is available online.
Published June 4, 2021