News Story
Four BIOE Ph.D. Students Awarded NIH Fellowships
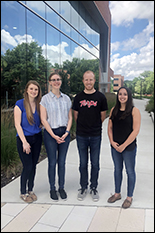
From left: Emily Gosselin, Mary Doolin, John Daristotle, and Michelle Bookstaver
Fischell Department of Bioengineering (BIOE) Ph.D. students Michelle Bookstaver, John Daristotle, Mary Doolin, and Emily Gosselin were recently named recipients of the Ruth L. Kirschstein Predoctoral Individual National Research Service Award (F31) by the National Institutes of Health (NIH). Together, their NIH F31 fellowships could total up to $235,000 in funding over two years to support research in vaccine design, sprayable surgical sealants, idiopathic pulmonary fibrosis, and new therapies to treat multiple sclerosis.
Vaccines have reduced the public health threat of many infectious diseases, including polio, measles, mumps, and small pox.
They typically work by exposing the body to antigens – molecules that can trigger a specific immune response in the body. The antigens carried by vaccines mimic, or are fragments of, pathogens – a bacterium, virus or other microorganism that causes disease. Additionally, vaccines carry stimulatory signals – known as adjuvants –that help the immune system generate large responses against antigens. With both components, vaccines can mimic a specific pathogen in order to stimulate the body’s immune system to build up defenses against disease agents.
When it comes to tackling complex health challenges such as cancer, HIV, or Zika virus, however, vaccines must be fine-tuned to allow for more control over the specific features of the immune responses elicited.
“The heterogeneity and rapidly mutating nature of these diseases makes it particularly difficult to design effective vaccines for them,” BIOE graduate student Michelle Bookstaver said.
In recent years, bioengineers have intensely studied the use of biomaterials as vaccine carriers, as they offer advantages such as controlled release of the vaccine, targeting, and “cargo” protection.
As a member of BIOE Associate Professor and Associate Chair Christopher Jewell’s Immune Engineering Lab, Bookstaver worked with fellow researchers to develop a platform to self-assemble immune signals into nanostructured capsules. The materials mimic many of the attractive features of biomaterial carriers, but allow for a more simplified vaccine design because they are built entirely from immune signals.
To do this, the group created immune polyelectrolyte multilayers (iPEMs) composed entirely of disease-relevant antigens and stimulatory adjuvants. Because iPEMs incorporate key vaccine components at high densities, they enhance the body’s immune response and antitumor immunity.
Building on past findings, Bookstaver and fellow Jewell Lab members investigated how nanostructured assemblies of immune signals are taken up and processed to initiate adaptive immunity. With support from her NIH F31 fellowship, Bookstaver hopes to develop new strategies to assemble immune signals and build well-defined vaccines capable of changing the type and magnitude of the antigen-specific immune response.
In this way, she hopes to address one of the major limitations in vaccine design: the lack of simple, modular approaches to combat complex diseases.
"Today, vaccines are generally developed through a trial-and-error approach that requires significant resources and time,” Bookstaver said. “But, our hope is that future methodologies will allow selection of the immune signal combinations and formulation properties needed for a given vaccine or immunotherapy.”
Additionally, most vaccines being developed are made up of many complex parts such as antigens, adjuvants, stabilizers, carriers, attenuated pathogens, or other components required to increase potency.
"If we could develop well-defined adjuvants that target specific immune pathways, we could potentially tackle many of the inefficiencies we see with today’s vaccines,” Bookstaver said.
The Jewell Lab group hopes that new insights gleaned from their research could help speed design and translation of biomaterial carriers being considered for vaccination and immunotherapy.
Surgical Sealants
Researchers have long proposed the use of surgical sealants to replace the need for sutures. But, scientists have since struggled to develop an effective technique to apply these sealants to living cells or tissues.
To combat these challenges, BIOE graduate student John Daristotle and fellow researchers aim to develop a sprayable, biodegradable polymer surgical sealant for decreasing high mortality rate complications that occur after surgery. Daristotle is working with Department of Chemical and Biomolecular Engineering Professor and Chair Peter Kofinas (affiliate, BIOE, and Materials Science and Engineering) and Anthony Sandler, M.D., director of the Sheikh Zayed Institute for Pediatric Surgical Innovation at Children's National Health System, to use a technique known as solution blow spinning to deposit biodegradable polymer fiber surgical sealants to the site of surgery in vivo with exceptional sealing strength.
Because solution blow spinning can be used to fabricate nanofiber mats and meshes for precise and site-specific reconstruction in minutes, the technique could prove extremely useful in surgeries requiring the use of a hemostatic material or sealant. In fact, solution blow spinning is such a simple and transferable technique that bioengineers have successfully used commercial airbrushes—such as those typically used for painting—to generate nanofibers.
Additionally, this proposed technique is especially useful for instances in which conventional suturing may not be adequate, such as in cases of liver or lung resections. And, the application technique could prove invaluable to first responders to accident scenes involving significant injuries.
With support from his NIH F31 grant, Daristotle will work in Kofinas’ Functional Macromolecular Laboratory to improve the biocompatibility of polymer surgical sealants used in pre-clinical trials following intestinal anastomosis, a surgical procedure that connects two parts of the intestine after a portion of the intestine has been surgically removed.
The project will investigate different sprayable combinations of biodegradable polymers that can reduce inflammation or increase wet tissue adhesion. Building on previous work, Daristotle will use silica nanoparticles to improve adhesion and hemostasis – the process that causes bleeding to stop – at the site where the polymer surgical sealant is applied.
“The insights produced by these studies will also be applicable to other polymeric biomedical devices, such as sutures and tissue engineering scaffolds, increasing the significance of the work,” Daristotle said.
The group’s work was published in the May 2019 edition of Acta Biomaterialia. Daristotle served as first author and Kofinas as corresponding author of the paper titled, “Improving the adhesion, flexibility, and hemostatic efficacy of a sprayable polymer blend surgical sealant by incorporating silica particles.”
Pulmonary Fibrosis
Idiopathic pulmonary fibrosis (IPF) is a progressive lung disease that results in scarring of the lungs. Over time, the scarring worsens, making it difficult for the lungs to take in enough oxygen.
Little is known about what causes IPF, which affects more than 80,000 adults in the United States each year. There are no known cures and few treatment options for the disease, and the average survival time after diagnosis is just three years.
Fibroblasts are cells that produce extracellular matrix (ECM) – a complex meshwork of proteins and carbohydrates that determines the structure and function of tissue. In IPF, fibroblasts differentiate into what are known as contractile myofibroblasts. While fibroblasts produce ECM to maintain homeostasis and support cell function, myofibrolasts can severely impair lung function by causing excessive production of the ECM. When this happens due to IPF, it causes lung tissue to become stiffer, which leads to further progression of the disease.
As ECM production increases, the space in which cells exist becomes more confined. To date, scientists do not fully understand what role – if any – this confinement might play in fibroblast differentiation. But, advanced research in this area could shed new light on treatment options for IPF and other pulmonary diseases.
“The causes of IPF are not well known,” said Mary Doolin, BIOE graduate student and a member of BIOE Assistant Professor Kim Stroka’s Cell and Microenvironment Engineering Lab. “If we can even begin to scratch the surface by elucidating the mechanobiology of this disease, we may make a huge impact.”
Recently, researchers have explored the injection of mesenchymal stem cells (MSCs) or MSC secreted factors as a potential therapeutic for IPF. Outside the scope of IPF, scientists have investigated how MSCs – adult stem cells that can produce a variety of specialized cells such as cartilage cells, bone cells, and fat cells – could be used to treat certain diseases.
But, IPF would be a new frontier – and some studies indicate that current forms of MSC therapy could actually worsen IPF outcomes.
“MSC therapies are ill-understood yet are beginning to be used aggressively in clinics,” Doolin said. “There is a huge need to understand what these cells are doing before we can use them as safe, effective treatments for IPF.”
To advance understanding of how injection of MSCs or MSC secreted factors could impact IPF, Doolin and fellow members of the Stroka Lab are exploring two key questions. First, they want to see if increasing confinement experienced by fibroblasts could encourage differentiation into myofibroblasts. Second, they want to investigate if increasing confinement of MSCs could enhance their protective effects on fibroblasts and perhaps improve IPF outcomes by slowing the rate – or halting altogether – the process by which fibroblasts differentiate into myofibroblasts.
“Our hope is that we will improve understanding of IPF progression and, thus, improve methods of MSC culture for use in IPF treatments,” Doolin said.
Multiple Sclerosis
Multiple sclerosis (MS) is a debilitating disease that occurs when the body’s immune system incorrectly recognizes components of the central nervous system, causing inflammation and destruction of myelin, the fatty substance that surrounds and protects nerve fibers. When this happens, nerve fibers and cells are damaged, leading to a loss of motor function and other complications. According to the National Multiple Sclerosis society, the disease affects nearly 1 million adults in the U.S. alone.
“To lessen the immune system’s attack against the body’s healthy cells, conventional therapies often rely on general immune suppression – a strategy that has proven effective, but can leave patients susceptible to disease or infections,” said Emily Gosselin, also a member of Jewell's Immune Engineering Lab. Gosselin is working with fellow researchers to develop therapies that promote selective immune tolerance to myelin.
To do this, she and fellow researchers are looking to target treatments directly to the lymph nodes, the body’s immune system “command centers.”
Lymph nodes are packed with different types of immune cells. Many of these cells respond to a particular disease or infection. Fragments of viruses, bacteria, and tumors collected in the lymph nodes are presented to these cells as antigens – molecules that provoke a response to a specific threat. When an immune cell encounters the antigen it recognizes, the cell “activates” and multiplies. The immune signals these cells receive in combination with the antigen determines the cells function – either inflammatory or regulatory. These cells are then released into the blood and tissue to hunt their specific targets.
With support from her NIH F31 grant, Gosselin aims to develop therapies that induce antigen-specific regulatory cells (Tregs) capable of selectively controlling disease.
“Our hope is to design therapies for MS that knock-down myelin-specific inflammation, while leaving the rest of the immune system intact,” she said.
To meet this goal, she aims to use a lymph node injection technique to deposit polymer particles co-loaded with myelin self-antigens and an immunomodulator known as rapamycin in mouse models of MS.
The Jewell Lab has previously shown that a single injection of particles into the lymph nodes allows local programming of the lymph node microenvironment to promote Tregs, which permanently stopped and reversed disease-induced paralysis in a model of progressive MS.
Unfortunately, 85 percent of patients initially present with what’s known as relapsing-remitting MS – a form of the disease in which the immune system becomes reactive to different sections of myelin in the body over time.
This process of spreading – known as epitope spreading – complicates treatment regimens, and current therapies do not consistently work at all stages of disease. A relapsing-remitting model will allow for the study of how this type of MS progresses when treatment is administered at an earlier disease stage.
Preliminary data shows that treating during the first wave of relapsing-remitting MS with a single lymph node injection of particles co-loaded with rapamycin and the myelin epitope attacked during that wave can reverse disease and prevent relapse.
Moving forward, Gosselin plans to study how treatments at different stages of disease with different myelin epitopes alters disease progression and prevents relapse.
###
Bookstaver’s work with the Jewell Lab was supported in part by the U.S. Department of Veterans Affairs, the Damon Runyon Foundation, the National Science Foundation, and the Alliance for Cancer Gene Therapy.
Daristotle’s work with the Kofinas Lab is supported in part by a National Institutes of Health Research Project Grant (R01).
Doolin’s work with the Stroka Lab was supported in part by a Burroughs Wellcome Career Award at the Scientific Interface, the University of Maryland Research and Scholarship Award, and the University of Maryland Graduate School Summer Fellowship.
Gosselin’s work with the Jewell Lab is supported in part by the National Multiple Sclerosis Society, and a National Institutes of Health Biomedical Imaging and Bioengineering (NIH NIBIB) R01.
Published June 13, 2019